Curing the Incurable: Cancer
With cancer rates skyrocketing, it's time to shed some light on what we know.
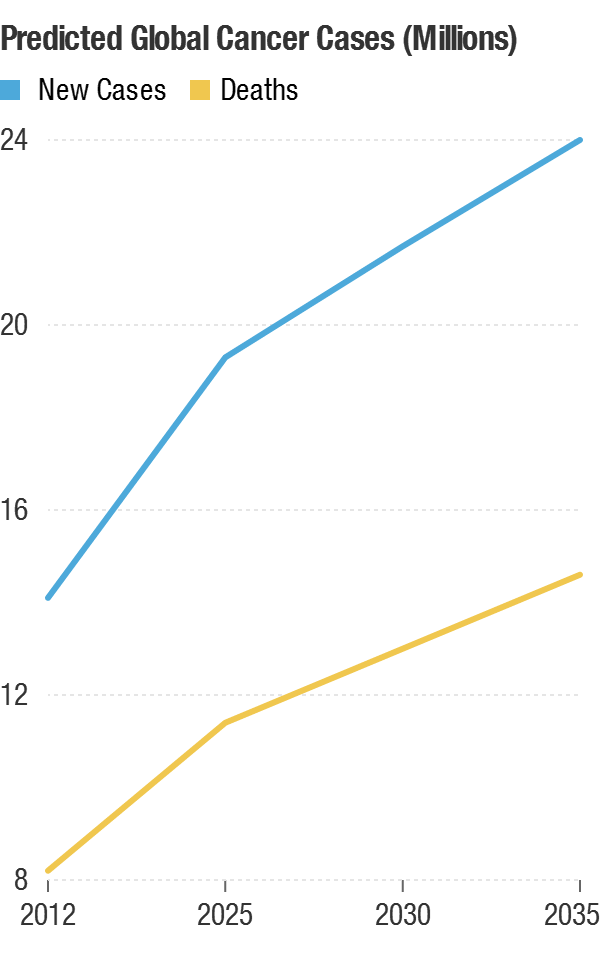
I’m a smoker. I’ve smoked a pack a day for almost 20 years. Knowing all I that do about health and medicine, you’d have to think I’m either crazy or suicidal, right? Why would I carry on smoking veritable cancer sticks, day in day out, knowing all we do about the risks?
I do not fear cancer. (Nor the other pathologies it can cause - but that’s a discussion for another day.) If I die of cancer one of these days, please feel free to put this right alongside my obituary and laugh at me, because it isn’t going to happen.
We have, through the efforts of tireless advocates and activists, brave practitioners, and the generally intelligent people that make up our wonderful world, come to understand that the public health apparatus is hopelessly corrupt. Billionaires like Bill Gates, the Rockefeller clan, and too many others to name, have long since taken control of the medical establishment: from the sworn public health officials like Fauci, to the formerly well-reputed journals like JAMA, and the hospitals that have now become the COVID killing fields; the entire medical system, from top to bottom, has been putting out falsified science, bad advice, and murderous protocols, alongside the ceaseless propagandizing against anything - at all - that might actually help. While their goals have ranged from simple profiteering, self-promotion and fame, to the furthering of the most pervasive and widely effected genocide the world has ever seen, the state of medical science is historically deplorable. Knowledge that was passed down for generations, hundreds of years, has been, of late, censored, buried and obliterated by the pioneers of so-called “modern medicine.” While I don’t plan to get too deeply into the history of it, the Rockefeller family - the same family which is now associated with the WEF, and bears responsibility for the COVID genocide as much as any other of their ilk - has played an instrumental role in the corruption of medicine over the last century.
One of the most insidious methodological switches that has been applied to modern medicine is a severe overreliance on statistical interpretations of trial data, to the exclusion of any significant consideration of proteomic interactions or mechanisms, which are instead relegated to curiosities of interest only to the scientists investigating them, with very little transference to the discovery and announcement of useful therapeutics. This switch has enabled the powers that be to lie with both statistics and impunity, concealing, discrediting and disallowing functional therapeutics that threaten either profits or the overarching genocidal agenda we find ourselves subjected to lately.
Proteomic investigation is what lead me to announce ivermectin to be curative for COVID-19, as early as April of 2020; proteomic investigation is what allowed me to predict, with extraordinary (and unfortunate) accuracy, many of the diseases now being suffered by the victims of the COVID vaccines; and now, proteomic investigation is the method I have applied here to reveal these cures for cancer, which we have been sitting on, while millions have died wholly unnecessary deaths, for decades. It was lamentable that I had so few avenues back then by which I could announce my discovery. Thanks to Twitter, I now have that means, and you’d best believe I’ll be using it.
With the revelations that the SARS-CoV-2 spike protein, as administered in the trillions via mRNA vaccines, exerts a carcinogenic effect on our cells, curing cancer has become rather a high priority. The new phenomenon of “turbo cancer” - previously perfectly healthy people who took a shot, and within weeks or months, find themselves with inoperable, metastatic stage 4 cancers - is only going to grow, as time goes on, and people continue blindly taking their boosters. They’ve given a large proportion of the world’s population a dizzying array of generally incurable diseases, cancers among them. Without cures for these conditions, we are entering a very dark age.
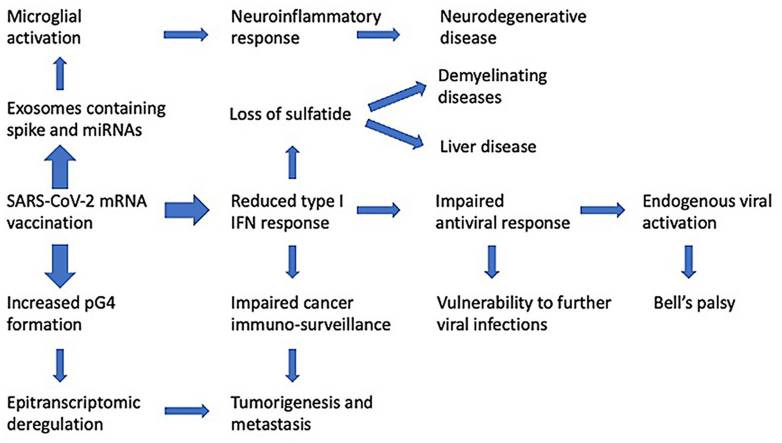
Fortunately, we have cures for many of these conditions. This article will be covering the cures for cancer. All cancers, regardless of how or why they came to be. If it sounds fantastical, you haven’t been paying much attention to the advancements that have been made over just the past few years: when you threaten the entire planet with bioweaponry, the entire planet’s going to start figuring out what to do about it. They’ve kept these things under wraps for long enough.
Without further ado: let’s get into it.
What is cancer, exactly?
The first step towards solving a problem is clearly identifying and characterizing it. There are a vast spectrum of different cancers, all of wildly varying etiologies, presentations, locations in the body, rates of progress, and immunological, metabolic and microbiological characteristics, many of which will change as the cancer progresses. There is also a distinction to be drawn concerning non-malignant tumors and growths.
Cancers are, simply, a disease of uncontrolled cell growth and division, wherein the various natural processes for containing them have partially or completely failed. There are many such processes, of varying efficacy; you may be surprised to know that we all have cancer! Cells become cancerous constantly, and both our cytosolic immune systems and wider humoral and innate immune systems generally obliterate them before we even notice, with an incredibly complex homeostatic system of checks and balances. It’s only when a cascading failure of these systems has occurred, due to innumerable potential etiologies ranging from radiation poisoning, to heavy metal toxicity, to old age, that a cancerous growth is able to take root and become pathogenic.
Read that again: We all have cancer, all the time.
So, what’s the difference between you or I, with no pathogenic cancers of which we’re aware, and a cancer victim withering away in hospice? Why are we fine, while others are not, and why have per-capita cancer rates skyrocketed over the last few decades?
What is cancer?
Cancer is a cascading series of failures of cytosolic and immune homeostasis which presents as uncontrolled tumor growth.
Important note: Homeostasis has absolutely nothing to do with homeopathy. Nor does the classification of homo sapiens have anything to do with homosexuality, for instance. Homo is a word, generally meaning “same,” with numerous and varied applications. You are not about to read some crystal-crunching pseudoscience.
As I have previously noted, we have four cures for cancer. For any given cancer, a combination of these therapeutics will effect a curative action.
These four cures are as follows:
Let’s explore how and why these four therapeutics are our most powerful weapons against cancer.
A suggested protocol, with several important caveats, is included at the end of the article.
Immune & Cellular Homeostasis
If you read my last major article, I introduced you to the basics of the immune system, and the concept of separating out cytosolic immunity as a class all its own, as opposed to the general characterization of simple innate versus adaptive immunity. That distinction, whilst relevant to understanding the IgG4 class switch, is indispensable and vital to gaining a thorough understanding of cancer. If you haven’t already read “the long version” of that article, I strongly suggest doing so before proceeding. I will be building upon some of the concepts covered there.
There are a numerous, but finite, number of pathways by which our bodies keep cancers in check. Our very existence is founded on mitosis, the reproductive division of cells; as with everything else in these fantastic biological machines we call home, our continued existence is the maintenance of a fine balance between life and death, and the slightest disruptions to that balance can cause a chain reaction, leading to - among many other things - cancer.
How do we normally keep cancer in check?
Cytosolic Immunity
A cell’s first line of defense against becoming cancerous is apoptosis. Apoptosis is the deliberate suicide of a cell: also applicable to numerous other pathologies, if it determines that it has become too badly damaged to recover, cancerous, or suffered any of a number of other insults, several pro-apoptotic cascades can become activated, culminating in the physical destruction of the cell’s vital proteins and organelles. These pathways are kept in check by anti-apoptotic factors; these two systems function in balance, and when one or the other becomes dominant, the cell either apoptoses, or resists apoptotic signals. The relevant pathways are as follows.
The p53 pathway. The p53 pathway is activated by sensor kinases, including ATM and Chk2, which monitor the cell’s DNA for damage or errors. Upon detection of damage, they phosphorylate the nuclear localization factor of the p53 tumor suppressor protein, allowing it to translocate to the cell nucleus and begin expressing p21, p16, p15, p19; this activates the cell cycle arrest pathway, initiates DNA repair, and preventing cell division. If the repair is deemed to have failed, BAX, BAK and/or PUMA are expressed, among others, initiating the mitochondrial caspase cascade, physically cutting up the cell’s vital organs to initiate apoptosis. Each of these steps is crucial to move the apoptotic pathway forward, and a failure at any stage may cause the cell to become cancerous.
Harris, S. L., & Levine, A. J. (2005). The P53 pathway: Positive and negative feedback loops. Oncogene, 24(17), 2899–2908. https://doi.org/10.1038/sj.onc.1208615 The G1/S checkpoint. In the normal cell cycle, the G1/S checkpoint serves as the regulatory phase prior to DNA replication. When a stress signal, such as oxidative stress, is detected, it activates the MAPK pathway via receptor activation and downstream signal transduction. The MAPK pathway, including components such as RTK, RAS, RAF, and MEK, leads to the phosphorylation and activation of MAPK, which in turn phosphorylates its downstream targets. These targets, including p38, JNK, and ERK1/2, then mediate cell cycle arrest and/or apoptosis by regulation of genes involved in cell division, such as Cyclin-Dependent Kinase Inhibitors (CKIs) and targets that lead to the activation of caspase-3, which then carries out the final process of apoptosis, respectively. This checkpoint is crucial in ensuring DNA integrity and preventing the progression of potentially damaged cells into mitosis. Failure at any stage of this pathway may result in the development of cancer. Furthermore, overactivation of the pathway, frequently observed in cancerous cells, causes the opposite effect: by phosphorylating Bcl-2, the protein acts at the mitochondrial membrane to inhibit the release of pro-apoptotic factors, preventing the destruction of the cell despite other pathways’ production of pro-apoptotic signal transducers.
Goldsmith, Z. G., & Dhanasekaran, D. N. (2007). G protein regulation of MAPK networks. Oncogene, 26(22), 3122–3142. https://doi.org/10.1038/sj.onc.1210407 The Hippo pathway. The Hippo pathway is a conserved signaling pathway that regulates organ size and tissue homeostasis by controlling cell proliferation and apoptosis. The core components of the Hippo pathway include the transmembrane receptor proteins MST1/2, the protein kinases LATS1/2, and the transkriptional co-activators YAP/TAZ. When the pathway is active, MST1/2 phosphorylates LATS1/2, leading to phosphorylation of YAP/TAZ, which then leads to their degradation and suppression of such anti-apoptotic genes like Bcl-2 and survivin, and activation of Bax among others. This suppresses cell proliferation and promotes apoptosis, thereby preventing uncontrolled cell growth by activating caspases and inducing cell death. Dysregulation of the Hippo pathway is implicated in various human cancers, including liver, lung, and breast cancer, highlighting the importance of this pathway in maintaining tissue homeostasis and preventing cancer.
Zhao, B., Lei, Q.-Y., & Guan, K.-L. (2008). The hippo–yap pathway: New connections between regulation of organ size and cancer. Current Opinion in Cell Biology, 20(6), 638–646. https://doi.org/10.1016/j.ceb.2008.10.001 The TGF-β pathway. The TGF-β pathway plays a crucial role in regulating cell growth, differentiation and apoptosis. Upon binding to its cell surface receptors, TGF-β activates SMAD transkription factors, leading to the repression of anti-apoptotic genes and the activation of pro-apoptotic genes. Additionally, the pathway also regulates cell growth by inhibiting the activity of Cyclin-Dependent Kinases, leading to cell cycle arrest at the G1 phase. This pathway acts as a tumor suppressor by promoting apoptosis in abnormal cells and inhibiting the growth of precancerous cells. Defects in this pathway can lead to uncontrolled cell growth and the development of cancer.
Liu, S., Chen, S., & Zeng, J. (2017). TGF‑β signaling: A complex role in tumorigenesis (review). Molecular Medicine Reports. https://doi.org/10.3892/mmr.2017.7970 The Wnt signaling pathway. The Wnt signaling pathway plays a crucial role in the regulation of cell proliferation and differentiation. In normal conditions, Wnt signaling maintains the balance between cell proliferation and apoptosis to ensure healthy tissue growth. However, when the pathway is activated excessively or inappropriately, it can lead to the development of cancer. This causes the accumulation of beta-catenin, a protein that normally gets degraded in the absence of Wnt signaling, leading to its overproduction and activation of target genes such as c-Myc and cyclin D1 that promote cell proliferation and inhibit apoptosis via Caspase-3. Dysregulation of Wnt signaling can also result from mutations in genes such as APC, AXIN1, CTNNB1 (encoding beta-catenin), leading to the activation of this signaling cascade and promoting the formation of tumors.
Zhan, T., Rindtorff, N., & Boutros, M. (2016). Wnt signaling in cancer. Oncogene, 36(11), 1461–1473. https://doi.org/10.1038/onc.2016.304 The Notch signaling pathway. The Notch signaling pathway is a signaling mechanism that plays a role in cell differentiation, proliferation, and apoptosis. The pathway is initiated by the activation of transmembrane receptors Notch1, Notch2, and Notch3, by the binding of a Notch ligand on an adjacent cell. This causes translocation of Notch to the nucleus to activate transkription of CBF1 and MAML. These then drive the expression of target genes, including HES and HEY, which regulate cell fate decisions, including differentiation and survival. Disruptions in the Notch pathway, such as mutations in Notch receptors or ligands, can lead to the dysregulation of cell proliferation and differentiation, contributing to the development of cancer.
Nowell, C. S., & Radtke, F. (2017). Notch as a tumour suppressor. Nature Reviews Cancer, 17(3), 145–159. https://doi.org/10.1038/nrc.2016.145 The PI3K/AKT signaling pathway. The activation of the PI3K/AKT signaling pathway occurs when growth factor receptors on the cell surface bind to their ligands, triggering the activation of PI3K. PI3K then generates PIP3, which recruits the serine/threonine kinase AKT to the plasma membrane. Once activated, AKT phosphorylates and inhibits the activity of pro-apoptotic proteins, such as BAD and FOXO, leading to the suppression of their apoptotic function. AKT also activates mTORC1, which regulates cellular metabolism and promotes cell survival by stimulating the expression of anti-apoptotic genes Bcl-2 and Bcl-xL, and is also, critically, a key step in the cascade required for the production of inhibitory PD-L1. The stability of mutations in the PI3K pathway can be promoted by the chaperone protein hsp90, which would otherwise fail to produce inhibitory signals. PI3K/AKT overactivation is also partially induced by PD-L1, which in concert with a self-antigen presenting MHC complex, results in the destruction of otherwise useful CD8 cells, and the cross-reactivity between PD-L1 creates a positive feedback loop in which the production of anti-apoptotic signals continuously escalates.
Rascio, F., Spadaccino, F., Rocchetti, M. T., Castellano, G., Stallone, G., Netti, G. S., & Ranieri, E. (2021). The pathogenic role of PI3K/Akt pathway in cancer onset and drug resistance: An updated review. Cancers, 13(16), 3949. https://doi.org/10.3390/cancers13163949
Got all that?
Oh, don’t lie to me. While the nitty-gritty of it is extraordinarily complex, the bottom line is that through various means, various checkpoint pathways are either activated or deactivated, which cause cascading proteomic interactions, phosphorylations etc, constituting the cytosolic immune response pathways by which a cell responds to becoming cancerous, and cancer, as we know it, arises when one or multiple of either these checkpoints, or the pathways they activate, become damaged and inoperative. As hallmarks of cancerous growth, the specifics vary extremely widely.
However, what does not vary widely is the specific cell functions that lead to the eventual cytosolic immune-mediated apoptosis of a cancerous cell. Each cell is an individual, unique living organism, and cellular apoptosis is the end of the line for a cell; as such, the process is tightly regulated, and there is only a short list of cascade transduction signals which can lead to apoptosis. These are as follows:
Caspases
AIF (apoptosis inducing factor)
Endonucleases
Granzymes
Bid (BH3-interacting domain death agonist)
DR5 (Death receptor 5)
FADD (Fas-associated protein with death domain)
Thus, as is often the case in proteomic systems, we have two competing imperatives:
The imperative to destroy the cell, given a stimulus indicating the cell has become cancerous or damaged;
The imperative to protect the cell from apoptosis, in the absence of such a stimulus, or the presence of a stimulus indicating the cell needs to survive and defend itself (eg. activation of the NF-κB pathway.)
Failures of one or more apoptotic mechanisms, or overactivation of one or more anti-apoptotic mechanisms, are the primary etiologies and commonalities between every single type of cancer in existence. Such failures represent a cascade transduction imbalance between the two competing imperatives, usually caused by either a mutation to critical protein(s), or an inappropriate exogenous receptor stimulus affecting the cell. Notably, a vast majority are directly attributable to mutations, particularly in critical checkpoints such as PI3K; these mutations result in the ribosomal production of malformed proteins. Ordinarily, such proteins would be quickly degraded by the chaperone and proteasome systems, which are tasked with protecting the cell from translation errors. However, most cancers as we know them also have another curious feature: upregulation of heat shock protein 90 (hsp90) production. Heat shock proteins, as the name implies, evolved largely to protect cells from external, notably inflammatory, stressors; as most cancers are not immediately medically detected, this is highly likely to be due to the body’s immune response to detection of a cancerous cell, and the cell’s subsequent mutagenic evolutionary effort to survive the inflammatory onslaught of the immune system, permitted to carry on due to mutations in critical checkpoint cascade proteins. Without the stabilizing influence of hsp90, among others, such deformed proteins will otherwise be destroyed before they can induce aberrant signaling cascades.
This brings us to our first cure…
Ivermectin
Among other actions, Ivermectin binds to the hsp90 protein, and other relevant heat shock proteins, at the interface between the N-terminal ATPase and the middle domains, preventing the stabilization of mutant checkpoint proteins. Furthermore, ivermectin acts to suppress the action of TAMs, which otherwise produce aberrant cytokine signals that act to suppress tumor apoptosis via a number of the above mentioned pathways, particularly TGF-β, and also upregulates the expression of the p53 tumor suppressor gene.
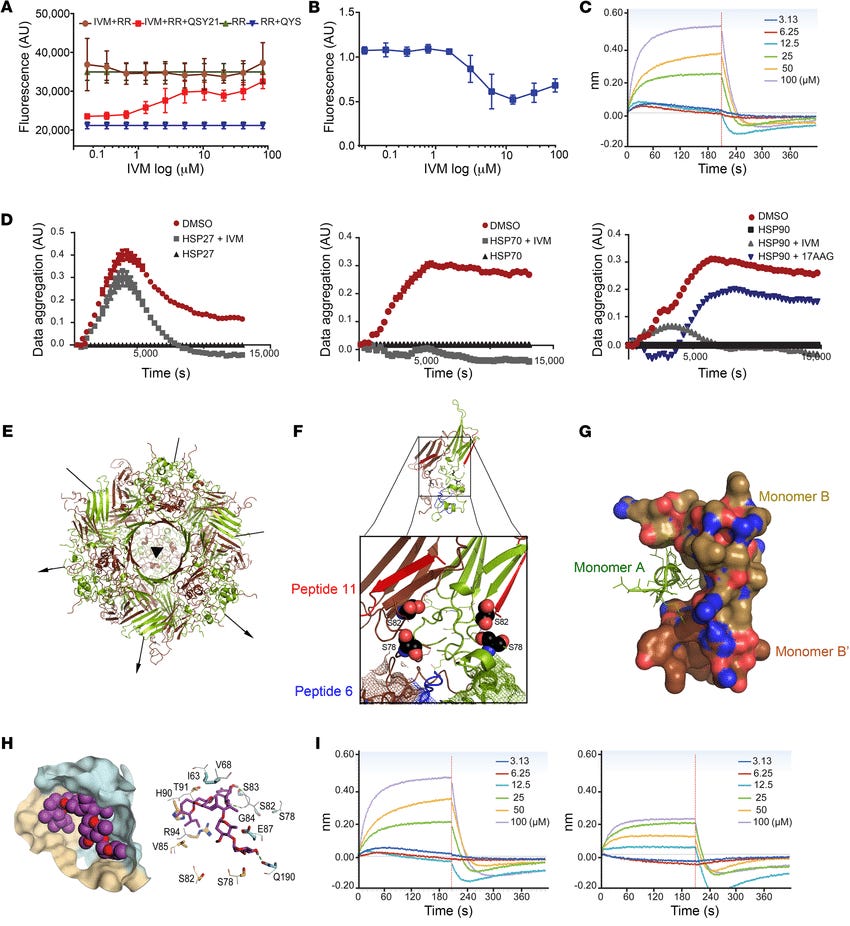
ATP, adenosine triphosphate, is the primary energy transporter which all biological systems, in all domains of life, require, in order to release the energy necessary to actuate the various mechanical functions of a cornucopia of proteins. Having lost the ability to bind ATP, the hsp90 chaperone is rendered nonfunctional, and malformed proteins are not stabilized. This action prevents the maturation and action of several mutant-form proteins involved in anti-apoptotic signaling, most notably the PI3K protein and pathway; without PI3K producing anti-apoptotic signals, the natural homeostatic process resumes, the mutations are detected, and the cancerous cells apoptose.
Of course, it’s never that easy. While ivermectin alone is capable of causing the apoptosis of a great many cancers, not every single cancer utilizes this pathway; anti-apoptotic signaling cascades also play an inhibitory role. Furthermore, owing to the relatively rapid replication rates of cancerous cells, and the evolutionary imperative toward survival, further mutations are often present across the mass of a tumor. Thus, of a given tumor mass, only perhaps 80% may be vulnerable to ivermectin; if that 80% is killed in this manner, then the 20% that remains will, by default, not be vulnerable to the correction of this pathway, and relapse occurs, with the remainder having become more difficult to treat. To begin to address further pathways, we now need to take a step back outside of the cell, and examine the relevant aspects of the adaptive and innate immune systems, their signaling pathways, and the various manners in which things go wrong.
The Immune System: Natural Killer Cells
Natural killer (NK) cells are the most relevant cancer-fighting cells of the innate immune system. Generally, rather than responding to a signal that some given stimulus means a cell is infected, or is a foreign pathogen, they default to killing any given cell they encounter, unless it presents a recognizable self-antigen that the NK cell is expecting. This is called the “missing self” pathway, and constitutes an effective inverse check: whilst other immune cells check for the presence of foreign antigens, NK cells generally check for the absence of self-antigens. To use a policing analogy: while most immune cells want to see evidence of a crime before they target an offender, NK cells wander the street and demand everyone’s papers, regardless of any evidence of a crime, and summarily execute anyone that fails to comply.
Due to the rapid rate of replication, mutations accumulate much more frequently in cancerous cells. Coupled with the overproduction of heat shock protein chaperones and upregulated function of the proteasome-ubiquitin system via various means, which permits cell survival in the face of extensive mutations to vital proteins, failures occur in the expression of the transporter associated with antigen processing (TAP) complex, and β2-microglobulin; these cause a loss of MHC-I self-antigen transport and surface presentation capacity, which causes an NK cell to destroy the cancerous cell.
However, again owing to the rapid rate of replication, cancerous cells are under heavy evolutionary pressure; cells that fail to express MHC-I self-antigens will be targeted and destroyed, whilst cells that happen to do so may not be targeted; as such, the immune system is presented with a hodgepodge of effectively random characteristics, some of which can be targeted for destruction, and some of which cannot.
While this permits the immune system to keep tumor growth in check, it also exerts heavy selection pressure on a tumor, and by only stripping away what effectively constitutes the weakest of the links in the tumor’s chain, it leaves behind a dense core of cancerous cells, all sharing very similar characteristics and MHC-I complexes that make the tumor effectively invisible to elements of the immune system, and permits it to continue growing relatively unabated. While this is very bad news in general, it also opens up an avenue which permits very rapid clearance of the remaining tumor mass, upon proper treatment, via T-cell antigen recognition and activation.
CD8 and CD4 T-cells
T-cells play a vital role in recognizing and responding to abnormal cells, including cancerous and infected cells, in the immune system. T-cells possess T-cell receptors (TCRs) that allow them to bind MHC-I-peptide complexes on the cell surface, which determines whether an immune response will be initiated. If the TCR binds to a complex that indicates a healthy and functioning cell, or if costimulatory signals are absent, they will not initiate an immune response and will ignore the tumor.
To activate T-cells, the presence of neoantigens in the MHC-I-peptide complex, which are produced from mutated proteins, as well as the presence of costimulatory signals at the cell surface, is required. There is a long list of such signals:
CD70
CD28
CD80 (B7-1)
CD86 (B7-2)
4-1BBL
OX40L (CD134L)
CD27L
GITRL
CD30L (CD153)
CD40L (CD154)
LIGHT (TNFSF14).
However, absent some stressor, cancerous cells will generally not sufficiently express these costimulatory signals, in which case even the presence of neoantigens will be insufficient to cause T-cell activation. Through the malfunction of any of the above pathways, they can also overproduce cytokines which disable the immune response and destroy effector T-cells.
We need an ace in the hole. We need something that can broadly disrupt the cells’ metabolism, doing so to degree sufficient to cause the production of some of these stressor chemokines at the cell surface. Further disruption of the cytosolic environment within the cell, leading to greater production of misfolded, malfunctioning proteins, and interrupting the cell cycle, is also very helpful.
This leads us to our second and third cures: fenbendazole and sodium bicarbonate.
Fenbendazole
Fenbendazole exhibits numerous actions against cancerous cells. Most relevantly, it modulates the typically overactivated MAPK pathway, switching it to activate the apoptotic pathway, rather than the anti-apoptotic pathway; it also destabilizes microtubules, structural proteins required to maintain a cell’s integrity during the process of mitosis, among other functions; it also interferes with cancer cells’ glycolysis-dependent metabolism, upon which most cancers are heavily preferentially dependent, as well as functioning as an inhibitor of mitochondrial oxidative phosphorylation, or OXPHOS, which reduces the residual energy available via the ordinary metabolic ATP production pathway. This presents four unique pathways, which whilst not all applicable to all cancers, covers a very wide proportion, and induces metabolic crisis in a sufficient number of them to trigger the next cascade.
The detection of metabolic stress by such sensors as AMPK or mTOR causes the phosphorylation of the FOXO and NF-κB transkription factors, which enter the nucleus and produce a number of chemokine signals to alert the body to the cell’s condition. Among them is CD80, a vital costimulatory signal that, in concert with TCR binding to an MHC-I-neoantigen complex, activates CD8 T-cells (as opposed to a self-antigen binding, which will deactivate them), and may also activate otherwise inactive NK cells in the vicinity. The destruction of the cell, whilst perhaps not yet widespread or sufficient to constitute an eradication of the tumor, releases the cell’s contents into the bloodstream for antigen capture by dendritic cells. Both healthy self-antigens and neoantigens are captured; self-antigens are ignored, while neoantigens are presented to CD8, CD4 and B-cells, along with further activating chemokines. Given that the natural, unaided immune response heretofore has narrowed down the morphology of the remaining tumor mass to a (relatively) uniform, immune evasive conformation, this step enables the broad recognition of that core tumor mass: where previously it was invisible, now the neoantigen complexes presented on the surface constitute a flashing beacon, which the immune system can now recognize and destroy. The presence of a large mass of newly recognizable antigen causes a much stronger, nonspecific response in the area of the tumor; this overwhelming inflammatory response will cause significant collateral damage, destroying the recognizable tumor cells, otherwise unrecognizable cells in close proximity, and likely a not-insignificant number of entirely healthy cells also in the vicinity.
Sodium Bicarbonate
Rumors have gone around for quite some time regarding the effect of an “alkaline diet,” the utility of alkalized water, or other such implications of alkalization on cancers. There have also been poorly conducted studies that have aimed to discredit the concept, which, coincidentally, happen to be the first results served up by Google upon a cursory search - in contrast, the very second result is a study showing quite the opposite. “There is no evidence” seems to be a very common refrain when the evidence is actually overwhelming. However, as with everything else, the extreme diversity of various features in various cancers generally precludes the possibility of any single drug or therapy functioning as a magic bullet. It is only through combination therapy that we are able to cover all the necessary bases in a manner which may be called broadly curative.
The concept behind the use of sodium bicarbonate is rather simple, making use of the Warburg effect: raising the extracellular pH reduces the ability of the cell to moderate the cytosolic alkali tumor environment, exacerbating metabolic stress across the totality of the tumor microenvironment. Metabolic stress induces similar chemokine production to that described above, and the rest is history, inducing the same immune cascades. However, without simultaneous treatment with other elements of the protocol, alkalization alone will drive evolutionary pressure to blunt the effect, and rather than acting to cure the patient, will simply cause various characteristics of the cancer to change, merely extending life expectancy, rather than completely obliterating the cancerous mass.
The precise amounts necessary to induce sufficient alkalization remain a mystery; however, as simple dietary changes have been demonstrated to significantly extend life expectancy, it is reasonable to assume that in combination with other mentioned therapeutics, the change does not need to be particularly large.
Moving away from alkalization, let’s explore the fourth cure:
Ascorbic Acid
Ascorbic acid, or vitamin C, has a rich history and diversity of function; to date, my favorite application is against infectious disease, wherein it effectively supercharges neutrophil phagocytosis, reducing inflammatory NETosis and rapidly reducing serum viral loads to zero. However, it also exhibits a different action against numerous cancers.
Cancers which preferentially rely on glycolysis require an enhanced capacity for glucose uptake. This is achieved via the upregulation of GLUT1 glucose transporters presenting on the cell surface. However, GLUT1 also freely transports dehydroascorbic acid, the oxidized form of ascorbic acid, into a cell. As we no longer endogenously produce vitamin C, this is ordinarily a tolerable circumstance; much more glucose is adsorbed into the cell for metabolism, and the small quantity of ascorbic acid is useful for various other metabolic functions. However, in very large quantities, ascorbic acid, and the reduced form DHA (dehydroascorbate) induces extreme oxidative stress within the cancerous cell; if that stress is insufficient to outright destroy the cell, that oxidative stress triggers the production of numerous cytokines, including our new best friend CD80, which helps to initiate the CD8-related cascade described above.
Of course, not all types of cancers are vulnerable to this pathway, and it is also directly neutralized by sodium bicarbonate. As a very powerful anti-inflammatory immunomodulator, it may also interfere with the inflammatory response required to target a large tumor. For this reason, a decision must be made as to which of the two is the more appropriate, considering the characteristics of the specific type of cancer being treated, and they should not be used simultaneously. The slight alkalization caused by sodium bicarbonate will not last particularly long; for that reason, both sodium bicarbonate and ascorbic acid can be used in an alternating fashion to maximize the effects of both treatments.
Synthesis
We have described four therapeutics by which, when used in conjunction, cancerous cells can be caused to undergo both apoptosis and loss of immune evasive features to cause subsequent attack by the immune system. These pathways, broadly summarized, are as follows: